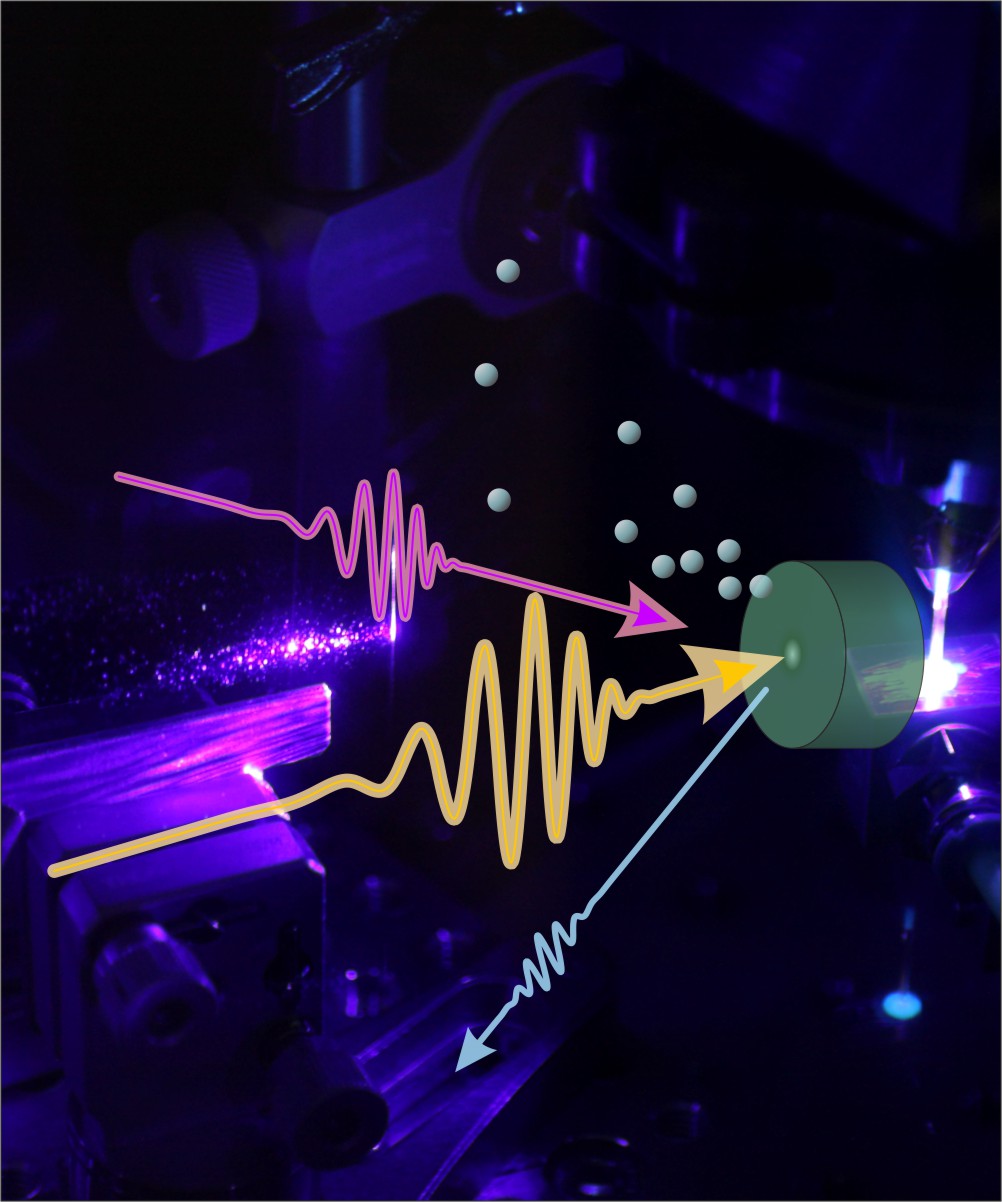
We investigate solids, interfaces, surfaces and molecules with high spatial and/or time resolution by using a variety of methods. Some of these methods are described in this section.
Angle-resolved photoemission spectroscopy (ARPES) provides priceless information about the quantum nature of electrons inside solids. Extending this method into the ultrafast time domain, time- and angle-resolved photoemission spectroscopy (trARPES) and two photon photoemission (2PPE) spectroscopy employ a combination of two femtosecond (10-15 s) laser pulses to additionally access dynamical properties. The femtosecond time resolution is achieved by the variation of the time delay between pump and probe pulse: The pump pulse creates a non-equilibrium distribution of electrons in the sample and the resulting relaxation dynamics in the intermediate state, defined by interactions in the solid, are monitored by the time-delayed probe pulse.
In trARPES, high energy (XUV) probe pulses are used to gain access to a wide area of the Brillouin zone. In 2PPE spectroscopy, both pump and probe laser pulses have energies below the sample work function (Φ), providing a background-free mapping of the unoccupied band structure. In both cases, first a photon of the pump pulse is absorbed by an electron below the Fermi level EF of the sample into an excited state. The photoemission occurs when the same electron absorbs a photon of the probe pulse, which excites the electron above the vacuum level Evac.
Analyzing the excited state’s time and energy scales provides important information on carrier relaxation pathways and coupling to other degrees of freedom. In addition, trARPES and 2PPE spectroscopy provide a sensitive tool to study optical control of electronic states, such as photoinduced phase transitions and hidden phases not accessible in equilibrium. We apply this method to a variety of materials, including novel 2-dimensional semiconductors and semimetals, interfaces, molecular systems and strongly correlated materials.
A diffraction image of a crystal taken with an ultrashort pulse of electrons or x-rays provides a snapshot of the material’s momentary atomic structure. We use femtosecond electron pulses in a time-resolved diffraction experiment to record a movie of the structure and motion of the atoms after a short laser pulse deposits energy in the material. In simple words, this experimental technique can be considered as a superfast thermometer measuring the temperature of the crystal’s lattice. The diffraction images contain elastic as well as inelastic scattering signals, which provide detailed information on the vibrational excitations (phonons) and their dynamics. In addition, the flow of energy between electrons and the lattice as well as between different types of phonons can be inferred. We apply this technique to a broad range of inorganic and organic nanoscale solids as well as heterostructures composed of multiple materials.
A broad variety of fundamental excitations in solids and molecules occur on THz ( = 10-12/s) time and energy scales. Among them are e.g. molecular and lattice vibrations (phonons), collective spin waves (magnons), Cooper pairs in high-temperature superconductors and internal excitations of bound electron-hole pairs (excitons). To directly access these quasi-particles in their natural ground or excited states, we generate resonant electromagnetic fields in the form of ultrashort laser pulses in the THz and MIR frequency range (0.1 THz – 40 THz), corresponding to 0.4 – 160 meV photon energy. The oscillation periods of these THz pulses (25 fs – 10 ps) also cover typical time scales of charge carrier scattering, electron-phonon equilibration or structural transitions, and can be therefore used as non-resonant, contact free bias fields. In the Department of Physical Chemistry, we employ the following three approaches to transiently observe or specifically control these ultrafast phenomena via phase-stable THz electric fields:
1. THz probing
Time-domain probing of THz fields in amplitude and phase (electro-optic sampling) provides direct access to the transient evolution of material properties such as complex valued dielectric function, refractive index and frequency dependent conductivity.
2. THz emission
Resolving the weak THz emission of accelerated charges provides a contact free and ultrafast Ampere-meter to measure currents during carrier transport, during trapping processes or during spin-charge conversion.
3. Strong field THz source
Our tabletop strong-field THz sources with field strengths exceeding 1 MV/cm at 1 THz and 50 MV/cm above 20 THz allow for specific creation and control resonant of low-energy excitations. Extending these excitation schemes into the nonlinear coupling regime, enables us to even control non-IR-active (e.g. dipole forbidden) modes. Moreover, we are specifically interested in exploiting tailored THz electric fields to drive structural dynamics along desired pathways.
Vibrational spectroscopy is a powerful tool to reveal chemical structures and dynamics. However, the spatial resolution is restricted to be a few hundreds of nanometers to a few micrometers due to the diffraction limit. The rapid advancement of nanoscience and nanotechnology requires much a higher sensitivity and spatial resolution. We are developing nanoscale and even atomic-scale vibrational spectroscopy by combining state-of-the-art scanning probe microscopy with surface-enhanced spectroscopy that utilizes strong enhancement and confinement of light occurring in metallic nanostructures through localized surface plasmon excitation. Tip-enhanced Raman spectroscopy (TERS) uses “nanolight” generated at the apex of a sharp plasmonic tip. We have recently demonstrated tip-enhanced “resonance” Raman spectroscopy (TERRS) which has an exceptional high chemical sensitivity and spatial resolution, about ~1 nm. This technique will allow us to investigate chemical structures and dynamics of nano-materials and single-molecules on surfaces.
[1] S. Liu, et al. Nano Lett. 19, 5725 (2019).
We employ visible to infrared ultrashort laser pulses for photoexcitation of the STM. Subsequent gating of the tunnel junction by ultrabroadband single-cycle THz-pulses allows for the local probing of the photoexcited state, providing new insight into the atomic-scale variations of the dynamics of charge carriers. Moreover, the application of a quasi-static femtosecond voltage pulse via THz-pulse excitation of the tunnel junction allows for the ultrafast injection of electrons into otherwise inaccessible electronic states. THz-controlled femtosecond electron injection in the STM, thus, provides a tool for probing ultrafast electronic and optoelectronic processes on the nanometer scale and below.
Nanoscale imaging and spectroscopy is of increasing importance in a wide range of research fields over physics, chemistry, biology, and medical applications. With conventional optics the spatial resolution of optical microscopy and spectroscopy is limited to be a few hundreds of nanometers due to the diffraction limit. We try to overcome this limitation and to achieve even atomic-scale spectromicroscopy by combining state-of-the-art scanning probe microscopy with plasmonics. To this end, we have developed a sophisticated method to control “nanolight” that can be generated by localized surface plasmon resonance at the apex of a scanning probe microscope tip. The plasmonic properties can be manipulated by nanoscale structures of the tip and we use nano-fabrication technique with focused ion beam. As a simple demonstration, we have recently showed that plasmonic Fabry-Pérot resonance can be controlled by making a single groove on the tip shaft [1]. This technique in combination with low-temperature scanning probe microscopy has a great potential for studying the nature of nanolight and atomic-scale light–matter interactions as well as for applying to many types of scanning near-field optical microscopies, thus paving the way for novel nanoscale imaging and spectroscopy. Furthermore, spectral control of the intense near-field at the apex of plasmonic tips may open up new opportunities for the realization of coherent laser-triggered electron point sources for low-energy electron microscopy and holography techniques.
[1] S. Liu, H. Böckmann et al. Nano Lett. 19, 3597 (2019).
Many surface science problems are intrinsically inhomogeneous on various length scales. It would thus be highly beneficial to have experimental tools at hand that are not only surface-specific but additionally also offer high spatial resolution. The Lattice Dynamics group has recently developed a new microscope that combines surface-specific vibrational spectroscopy with subdiffractional spatial resolution by means of infrared-visible sum-frequency generation. We employ the FHI infrared free-electron laser (FEL) to resonantly drive surface vibrations, and then use a time-synchronized visible laser for up-conversion. Conveniently, the resulting sum-frequency signal lies in the visible, and can thus be imaged with conventional optics, providing an image resolution well below the diffraction limit at the infrared resonant wavelength. By employing a wide-field imaging approach, we can harvest the full power of the FEL without damaging delicate surfaces.
First experiments with a normal incidence configuration of the visible laser provide a glimpse at the vast future opportunities of our approach. There, we observed localized surface phonon polariton resonances in subdiffractional SiC nanostructures with a spatial resolution of < 1 μm imaged at 11 μm infrared wavelength. The group is developing the SFG microscope into a universal surface science microscopy end station at the FHI FEL beamline.
Second order vibrational spectroscopy is nowadays an indispensable tool in surface science. Due to the special selection rules of second order nonlinear processes these laser-based techniques (e.g. sum frequency generation spectroscopy) are for many samples interface specific and allow for non-destructively studying interfacial processes by exclusively probing the vibrational fingerprint of interfacial molecular species. Despite the success of these techniques the obtained nonlinear spectra often contain insufficient information to unambiguously interpret the spectroscopic results and to obtain a clear physical picture of the interfacial region under investigation. This deficiency originates from the high complexity of interfacial systems which typically goes beyond the presence of a simple interfacial atomic layer.
In order to grasp this complexity, we have recently developed a new spectroscopic approach that combines the simultaneous acquisition of the phase resolved sum (SFG) and difference frequency responses (DFG) of a sample in the time domain. With this method we increase the number of complementary spectroscopic observables which allows us to get deeper insight into the complex physical properties of interfacial systems. By exploiting the symmetry properties of these two nonlinear signals in combination with highly accurate phase resolution we are able to disentangle different signal contributions, study local field effects and investigate the subsurface transition zone where the physical properties of the bulk approach the properties of the interface.