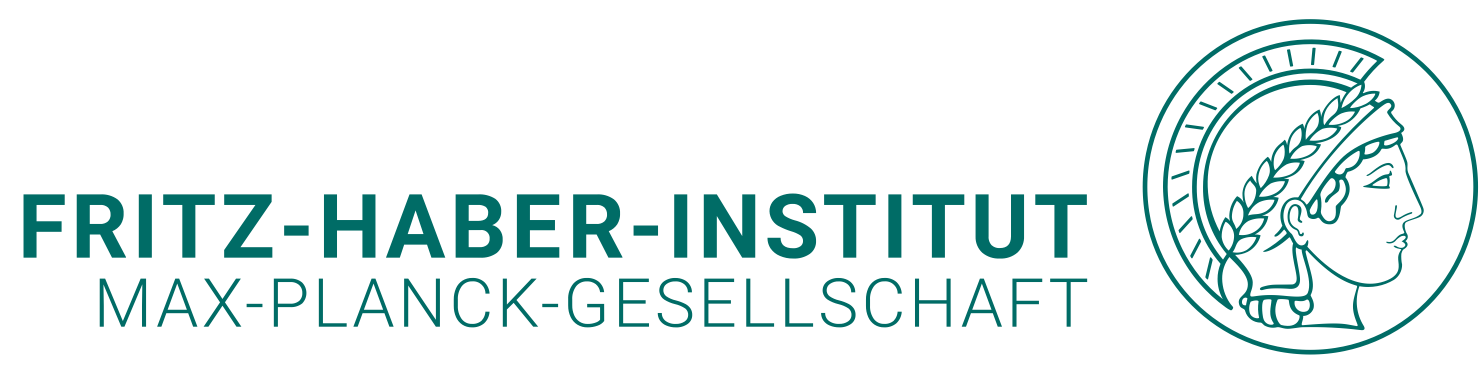
Physikalische Chemie - Direktor: Prof. Dr. Martin Wolf
Department Seminar
Host: R. Ernstorfer
Wednesday, May 30, 2018, 10:00 am
PC Seminar Room, G 2.06, Faradayweg 4
Tommaso Pincelli
Department of Physics, University of Milan
A spintronic oxide in the depth and time domain:
the insight of photoelectron spectroscopy
The advancement of technology requires pushing through the current limitations in terms of size, efficiency and switching speed of the elementary devices. One of the possible routes is the development of spintronics based on transition metal oxides (TMOs). The spatial limitations are overcome by manipulating both current flow and magnetic ordering within a single element, while dramatic variations in the transport properties (Metal-Insulator Transitions, MIT) arise from high-energy interactions (electronic correlation) allowing for fast switching mechanisms and efficient operation. Such a synthesis comes at a price, as the solid is brought very close to a quantum critical state, and thus responds to external perturbations in ways that are often difficult to interpret and measure. In the approach presented here, the boundaries of photoelectron spectroscopy (PES) are expanded to explore how the electronic structure relaxes external perturbations both in the depth and time domain.
The hole-doped rare-earth manganite La0.66Sr0.33MnO3 (LSMO) is chosen as a prototypical material for TMO spintronics. It displays phenomena such as colossal magnetoresistance and half-metallicity [1]. Optimally doped LSMO thin films can be used to inject fully spin polarized currents (the spin polarization at the Fermi level reaches about 100% for T<TCurie [2]) and ferromagnetic order persists up to about 350 K [3].
Such properties have been observed [4-5] to be suppressed by the presence of a crystal interruption, as the electronic structure relaxes the surface/interface perturbation with an intrinsic length scale (critical thickness). To disentangle the effect of intrinsic or extrinsic mechanisms, it is necessary to employ on every individual sample a quantitative, non-destructive technique with a high degree of control on the probing depth. In the approach described here, PES at various photon energies (spanning the soft to hard x-ray range) is applied for the first time to a quantitative assessment of the critical thickness [6]. We study the Mn 2p core level and, in particular, the bulk-only screening satellites related to the metallic and ferromagnetic state in LSMO [7-10], finding a critical thickness of 4 nm.
In the real-time domain, the discovery of unexpectedly fast magnetic phenomena has renewed interest in the study of non-equilibrium magnetic states in spintronic materials, including manganites [11-12]. The dynamics after ultra-short photoexcitation is explored by combining pump-probe magneto-optical techniques with pump-probe hard x-ray PES. The former is used to observe the evolution of the macroscopic order parameter, while the latter is able to detect the out-of-equilibrium state of the electronic configuration. Both techniques observe variations on long timescales in excess of 100 ps, a specific signature of the magnetic dynamics of half metallic ferromagnets [13]. This suggests that the coupling between magnetic order and half-metallicity of the electronic structure does not break down at ps timescales, bottlenecking the dynamics of the solid.
Finally, a description of the SPRINT laboratory is given, where a high harmonics generation (HHG) laser source is combined with advanced instrumentation [14-15] to perform time-resolved Extreme Ultraviolet PES experiments on magnetic materials.
[1] Y. Tokura et al. J. Magn. & Magn. Mater. 200, 1 (1999).
[2] J.-H. Park, et al. Nature, 392, 794 (1998).
[3] K. Horiba et al. Phys. Rev. B 71, 155420 (2005).
[4] J. H. Park et al. Phys. Rev. Lett. 81, 1953 (1998).
[5] A. Verna et al. JMMM 322, 1212 (2010).
[6] T. Pincelli et al. Nature communications 8, 16051 (2017).
[7] K. Horiba, et al. Phys. Rev. Lett. 93, 236401 (2004).
[8] J. Fujii et al., Phys. Rev. Lett. 107, 187203 (2011).
[9] Taguchi M. and Panaccione G., in Hard X-ray Photoelectron Spectroscopy, Springer. pp. 197-216
[10] Offi F. et al. Phys. Rev. B 75, 014422 (2007).
[11] E. Beaurepaire et al. Phys. Rev. Lett. 76, 4250 (1996).
[12] A.I. Lobad et al. Appl. Phys. Lett. 77, 4025 (2000); K. Matsuda et al. Phys. Rev. B 58, 4203 (1998).
[13] G. Müller et. al. Nature Materials 8, 56 (2009).
[14] T. Pincelli et al. Rev. Sci. Instrum. 87, 035111 (2016).
[15] T. Pincelli et al. Journal of Synchrotron Radiation 24, 175 (2017).
The hole-doped rare-earth manganite La0.66Sr0.33MnO3 (LSMO) is chosen as a prototypical material for TMO spintronics. It displays phenomena such as colossal magnetoresistance and half-metallicity [1]. Optimally doped LSMO thin films can be used to inject fully spin polarized currents (the spin polarization at the Fermi level reaches about 100% for T<TCurie [2]) and ferromagnetic order persists up to about 350 K [3].
Such properties have been observed [4-5] to be suppressed by the presence of a crystal interruption, as the electronic structure relaxes the surface/interface perturbation with an intrinsic length scale (critical thickness). To disentangle the effect of intrinsic or extrinsic mechanisms, it is necessary to employ on every individual sample a quantitative, non-destructive technique with a high degree of control on the probing depth. In the approach described here, PES at various photon energies (spanning the soft to hard x-ray range) is applied for the first time to a quantitative assessment of the critical thickness [6]. We study the Mn 2p core level and, in particular, the bulk-only screening satellites related to the metallic and ferromagnetic state in LSMO [7-10], finding a critical thickness of 4 nm.
In the real-time domain, the discovery of unexpectedly fast magnetic phenomena has renewed interest in the study of non-equilibrium magnetic states in spintronic materials, including manganites [11-12]. The dynamics after ultra-short photoexcitation is explored by combining pump-probe magneto-optical techniques with pump-probe hard x-ray PES. The former is used to observe the evolution of the macroscopic order parameter, while the latter is able to detect the out-of-equilibrium state of the electronic configuration. Both techniques observe variations on long timescales in excess of 100 ps, a specific signature of the magnetic dynamics of half metallic ferromagnets [13]. This suggests that the coupling between magnetic order and half-metallicity of the electronic structure does not break down at ps timescales, bottlenecking the dynamics of the solid.
Finally, a description of the SPRINT laboratory is given, where a high harmonics generation (HHG) laser source is combined with advanced instrumentation [14-15] to perform time-resolved Extreme Ultraviolet PES experiments on magnetic materials.
[1] Y. Tokura et al. J. Magn. & Magn. Mater. 200, 1 (1999).
[2] J.-H. Park, et al. Nature, 392, 794 (1998).
[3] K. Horiba et al. Phys. Rev. B 71, 155420 (2005).
[4] J. H. Park et al. Phys. Rev. Lett. 81, 1953 (1998).
[5] A. Verna et al. JMMM 322, 1212 (2010).
[6] T. Pincelli et al. Nature communications 8, 16051 (2017).
[7] K. Horiba, et al. Phys. Rev. Lett. 93, 236401 (2004).
[8] J. Fujii et al., Phys. Rev. Lett. 107, 187203 (2011).
[9] Taguchi M. and Panaccione G., in Hard X-ray Photoelectron Spectroscopy, Springer. pp. 197-216
[10] Offi F. et al. Phys. Rev. B 75, 014422 (2007).
[11] E. Beaurepaire et al. Phys. Rev. Lett. 76, 4250 (1996).
[12] A.I. Lobad et al. Appl. Phys. Lett. 77, 4025 (2000); K. Matsuda et al. Phys. Rev. B 58, 4203 (1998).
[13] G. Müller et. al. Nature Materials 8, 56 (2009).
[14] T. Pincelli et al. Rev. Sci. Instrum. 87, 035111 (2016).
[15] T. Pincelli et al. Journal of Synchrotron Radiation 24, 175 (2017).