Nonlinear optical Spectro-Electrochemistry
A deep understanding of electrochemical processes generally requires a combination of electrochemical and spectroscopic methods. While electrochemical analysis can provide the potential, current and charge information, the spectroscopies can reveal the electronic and vibrational properties of the reaction intermediates or products. The combination is referred to as spectro-electrochemistry. Among the modern spectro-electrochemical techniques, vibrational spectroscopy such as Infrared absorption and Raman scattering are powerful in terms of providing structural and chemical information of interfacial species and have thus been widely employed for in situ spectro-electrochemical characterization. However, IR and Raman spectroscopies are not surface specific. When the surface and the bulk have similar chemical compositions, i.e. bulk solvents and anions adsorb to the electrode surface, it is very challenging to selectively probe the adsorbed species and discriminate from those of the bulk. Vibrational sum frequency spectroscopy (VSFS), based on the 2nd order nonlinear optical process, is intrinsically surface specific hence an excellent tool in probing buried interfaces. Our group employs VSFG to study fundamental processes in electrochemistry, whereby a majority of work has been done in collaboration with Interfacial molecular spectroscopy group of Dr. R. Kramer Campen.
Structure of electric double layer structure

Electric double layer is the key component of electrochemistry, where reactants, products, ions and solvents follow certain spatial distribution at the interface. Currently the most accepted model is the Gouy-Chapman-Stern model (left panel of Figure 1), in which the interface has been divided into three region: the inner Helmholtz plane, where solvent and specific anions adsorb directly to the electrode; the outer Helmholtz plane, where solvated cations present; and the diffuse layer, where ions follow Poisson- Boltzmann distribution. However, this model is depicted based essentially on the thermodynamic measurements, i.e. the electrocapillary curve, surface excess, differential capacitance, etc. The molecular level information is still insufficient. Many of the recent observations cannot be explained by this qualitative model. For example, why different ions can affect the efficiency and selectivity of CO2 reduction? To gain such information, we have to directly probe the molecules and ions in the electric double layer. This is what we are doing in our group. A few examples are shown in the right panel of Figure 1. With VSFS, we have successfully probed the bias dependent structure of water on gold electrode1, the chemical and physical nature of sulfate anions on Pt(111) surface2,3, and also the anisotropic properties of the polarizability of perchlorate at the air/solution interface where weak electric double layer present4.
Structure and chemistry of adsorbate under potential control
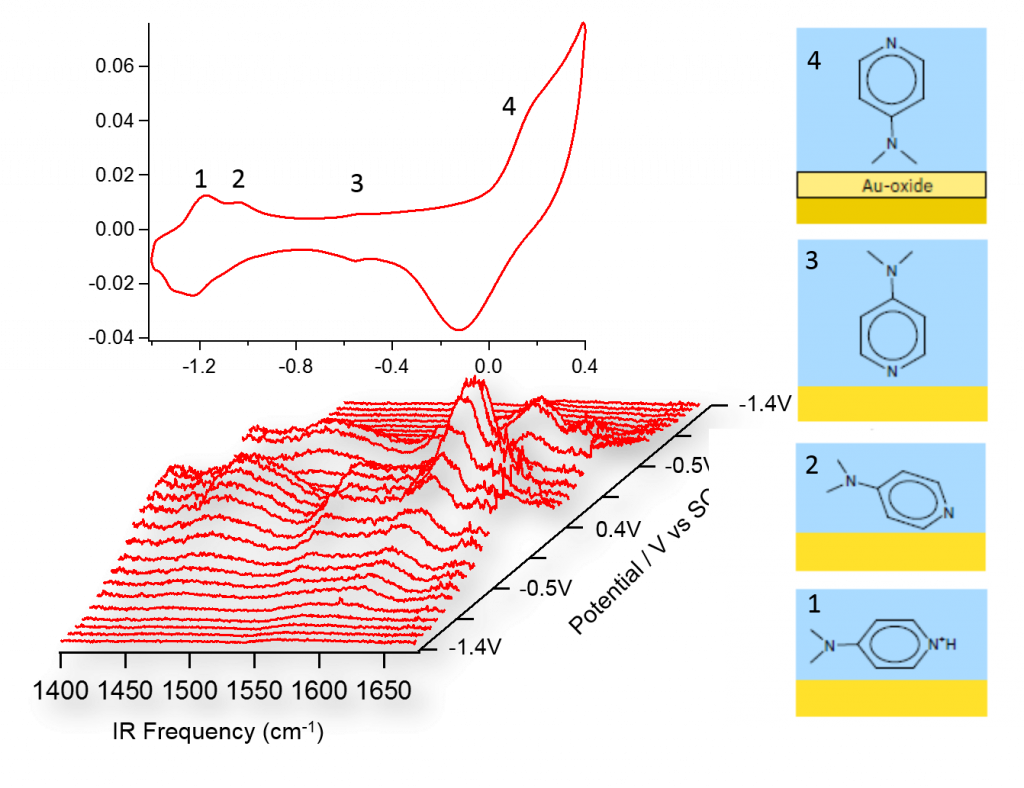
Electrochemistry is about the chemical reaction under bias control. In the ideal case, one would like to follow the evolution of all the structure and chemistry of the reactants and products during the reaction. Here we demonstrate with a model system of pyridine derivative (Dimethylaminopyridine) to monitor the deprotonation and phase transition processes with VSFS. As demonstrated in Figure 2, the spectroelectrochemical approach can provide fruitful information to reveal the detailed physical chemistry actions that happen as a function of bias.
Toward ultrafast electrochemistry
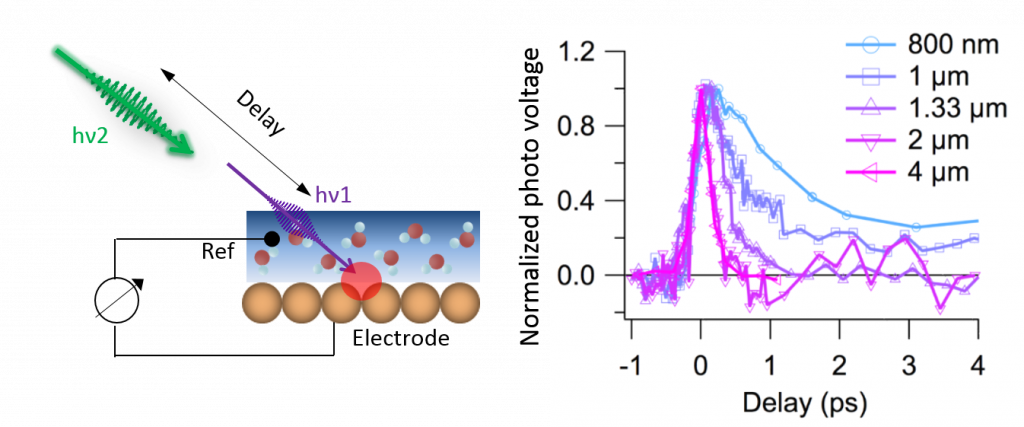
Many electrochemical and photo-electrochemical related processes take place on pico- or even tens of femto second time scales. However, a three electrode system coupled to a conventional potentiostat has a time resolution of, at best, 10 ns. To overcome this time resolution problem, we combined the photocurrent or photovoltage detection with ultrafast laser excitation. As demonstrated in the left panel of Figure 3, using a sequence of two ultrashort laser pulses of different photon energies and detection of the laser induced photovoltage as a function of the delay between the two incident pulses, ultrafast electrochemical processes on femtosecond timescales can be resolved. This method can be employed to study the evolution of the interfacial charge carriers which are directly relevant to photo electrochemistry. Since the measure quantity is charge instead of photon, the experimental geometry will be less restricted by the spectroscopic requirement, hence can be directly used for in situ characterization of the photovoltaic devices. Right panel of Figure 3 shows an example, where photo excited solvated electron at the gold solution interface is monitored. The trigger laser was chosen at 267nm. The probe laser (2nd pulse) was tuned from mid infrared to visible frequency region. The 2nd pulse induced photo voltage values as a function of delay are plotted in the right panel of Figure 3. Obviously, the dynamics are strongly dependent on the energy of the second pulse. Sub picosecond time resolution was achieved at the electrochemical interface. These probe energy dependent behaviors were understood to be related to the localization of the solvated electron at the interface.
References:
- Tong, Y., Lapointe, F., Thämer, M., Wolf, M., Campen, R. K. (2017) Hydrophobic Water Probed Experimentally at the Gold Electrode/Aqueous Interface, Angew Chem Int Ed, 56, 4211-4214.
- Zwaschka, G., Wolf, M., Campen, R. K., Tong, Y. (2018) A Microscopic Model of the Electrochemical Vibrational Stark Effect: Understanding VSF Spectroscopy of (bi)Sulfate on Pt(111), Surf Sci, 678, 78-85.
- Zhang, I.Y., Zwaschka, G., Wang Z., Wolf, M., Campen, R. K., Tong, Y. (2019) Resolving the Chemical Identity of H2SO4 derived anions on Pt(111) electrodes: They are sulfate, Phys Chem Chem Phys, 21, 19147-19152
- Tong, Y., Zhang, I. Y., Campen, R. K. (2018) Experimentally Quantifying Anion Polarizability at the Air/Water Interface, Nat Comm, 9, 1313.